The McNair Medical Institute at Baylor College of Medicine was established in 2007 to recruit talented scientists and physician-scientists from around the world to the Texas Medical Center. These McNair Scholars pursue collaborative and transformational research in the areas of breast and pancreatic cancer, juvenile diabetes and the neurosciences.
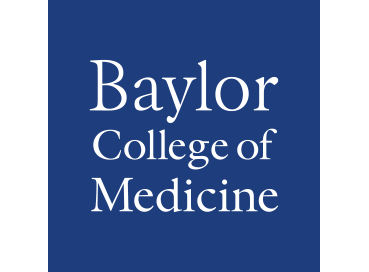
Qiancheng Zhao, Ph.D.
Assistant Professor of Endocrinology
June 2024
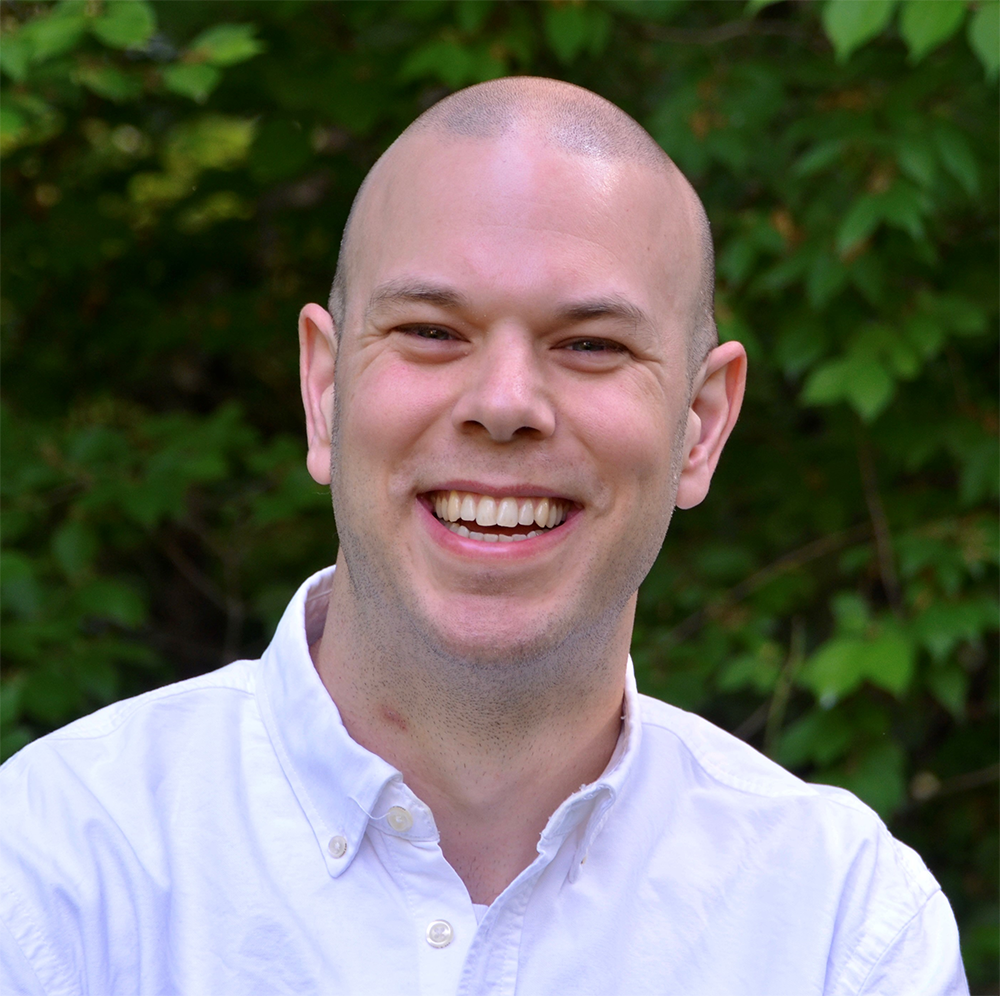
Dr. Michael Bround, Ph.D.
Assistant Professor of Integrative Physiology
March 2024
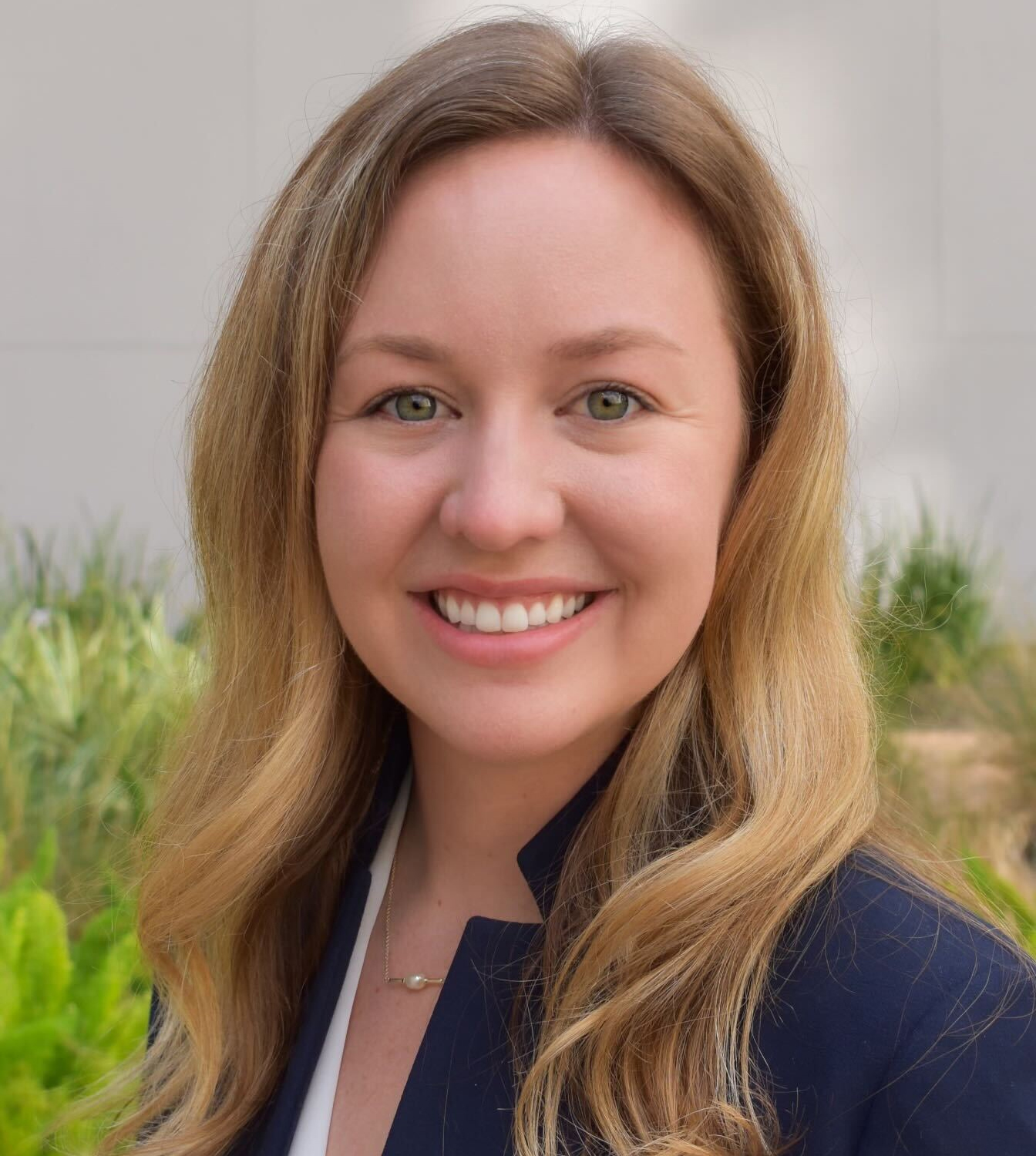
Dr. Nicole Provenza, Ph.D.
Assistant Professor of Neurosurgery
February 2024
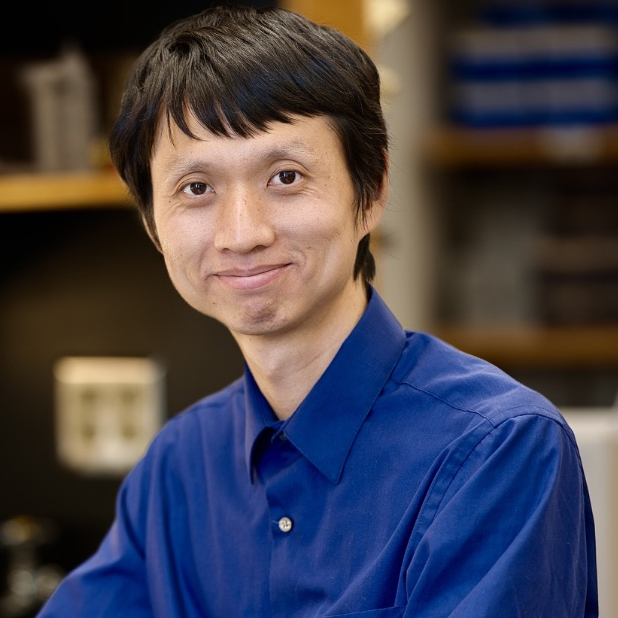
Yudong Gao, Ph.D.
Assistant Professor of Neuroscience
July 2023
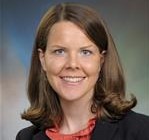
Shelly Buffington, Ph.D.
Assistant Professor of Neuroscience
May 2023
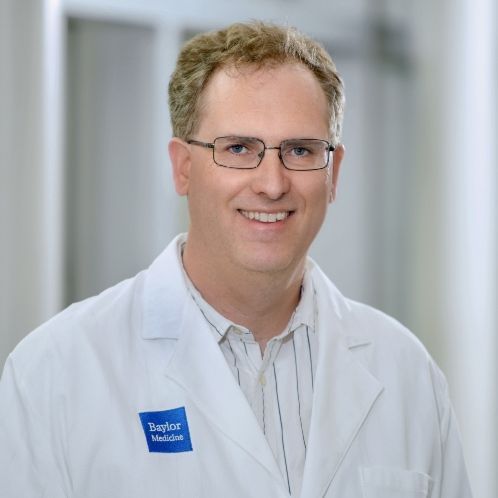
Benjamin Hayden, Ph.D
Professor of Neurosurgery
March 2023
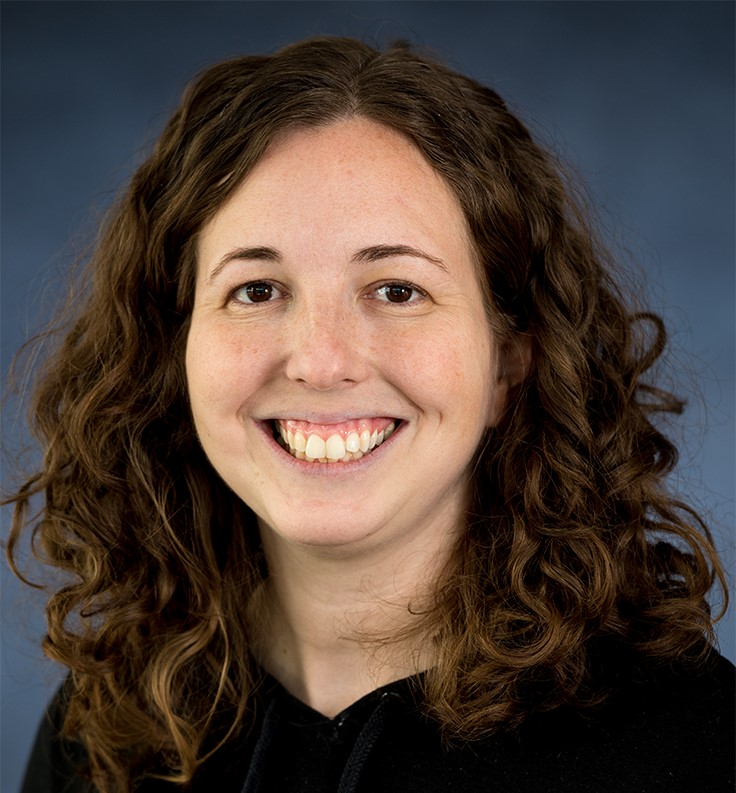
Sarah Heilbronner, Ph.D.
Associate Professor of Neurosurgery
March 2023
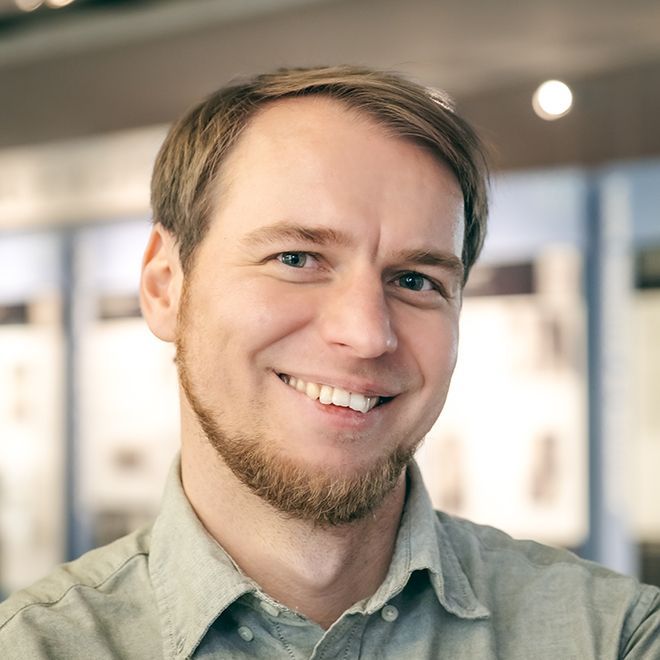
Barna Dudok, Ph.D.
Assistant Professor in Neurology
January 2023
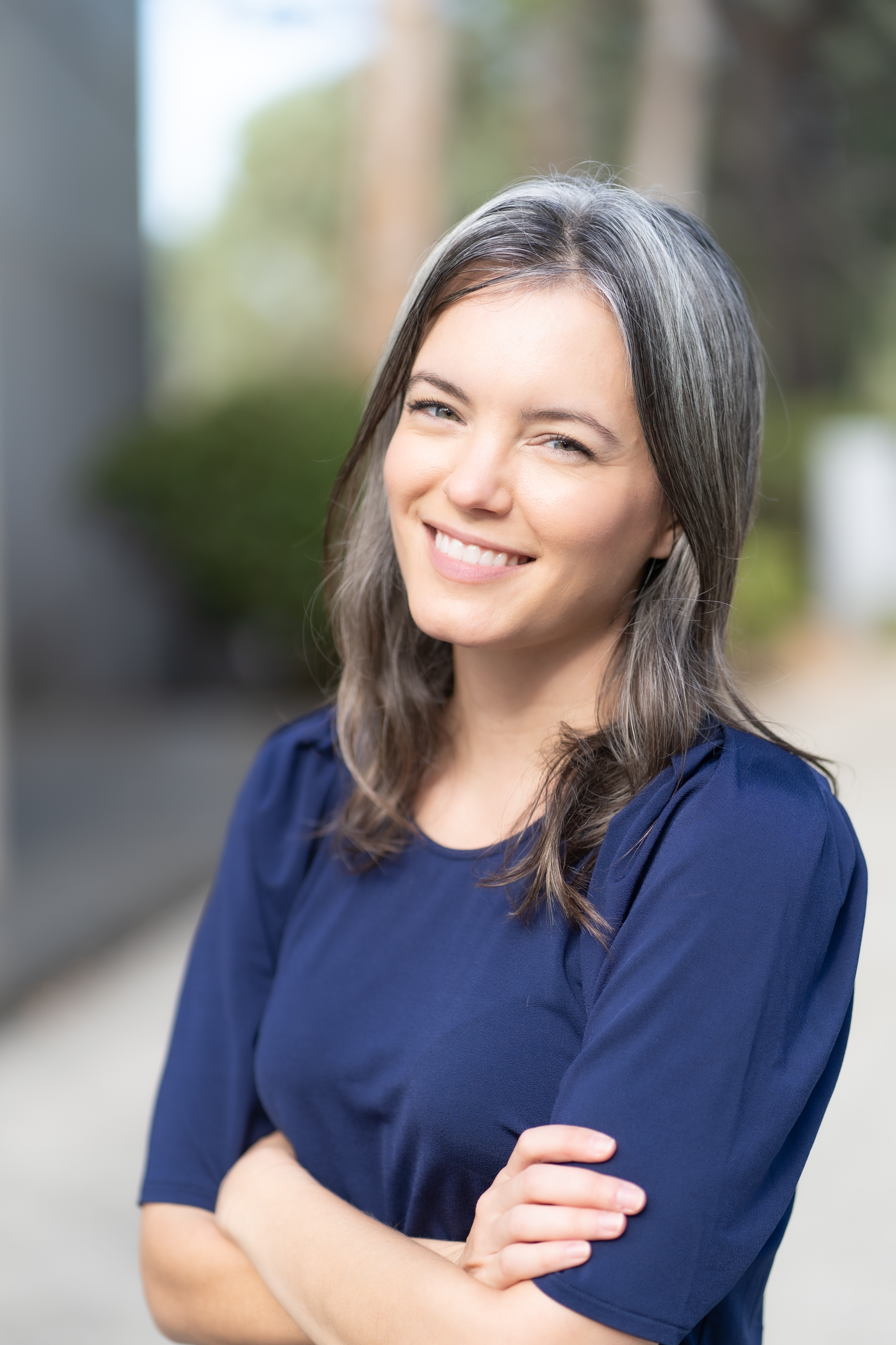
Kara Marshall, M.S. Ph.D.
Assistant Professor of Neuroscience
Jan and Dan Duncan Neurological Research Institute at Texas Children’s Hospital
June 2022
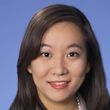
Hsiao-Tuan Chao, M.D., Ph.D.
Assistant Professor of Molecular and Human Genetics and Pediatrics
February 2019
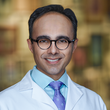
Sameer A. Sheth, M.D., Ph.D.
Associate Professor in Department of Neurosurgery
February 2018
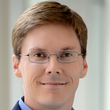
Thomas (Trey) Westbrook, Ph.D.
Associate Professor in the Department of Molecular & Human Genetics and Biochemistry & Molecular Biology | Program in Integrative Molecular and Biomedical Sciences and Program in Developmental Biology
May 2017
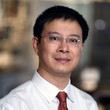
Bing Zhang, Ph.D.
Professor in the Department of Molecular and Human Genetics and the Lester and Sue Smith Breast Center
October 2016
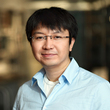
Nuo Li, Ph.D.
Assistant Professor of Neuroscience
April 2016
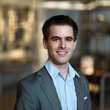
François St-Pierre, Ph.D.
Assistant Professor of Neuroscience
October 2015
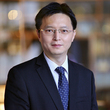
Chenghang Zong, Ph.D.
Assistant Professor of Molecular and Human Genetics
August 2013
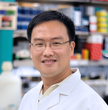
Xiang "Shawn" Zhang, Ph.D.
Assistant Professor of Molecular and Cellular Biology
Named June 2011
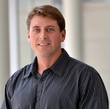
Benjamin Russell Arenkiel, Ph.D.
Assistant Professor of Molecular & Human Genetics and Neuroscience
December 2010
Emeritus McNair Scholars
- Erez Lieberman Aiden, Ph.D.
- Melissa Bondy, Ph.D.
- Malgorzata Borowiak, Ph.D.
- Matthew Ellis, M.D.
- Jake A. Kushner, M.D.
- Russell Scott Ray, Ph.D.
- Koen Venken, Ph.D.
These Scholars have reached Emeritus status and no longer have active McNair funding. They continue to make a great impact on behalf of the McNair Medical Institute and carry the McNair Scholar name.
In Memoriam
McNair Medical Institute at Baylor
Learn more about the McNair Medical Institute at Baylor College of Medicine and the support of Robert C. and Janice McNair.
Afraid of food? The answer may be in the basal forebrain
McNair Scholar Dr. Benjamin Arenkiel studies the way a small neuronal circuit in the basal forebrain connects with and overrides feeding behaviors regulated by the hypothalamus.
McNair Scholars talk about how the McNair Foundation supports research for a more promising tomorrow. Read more.