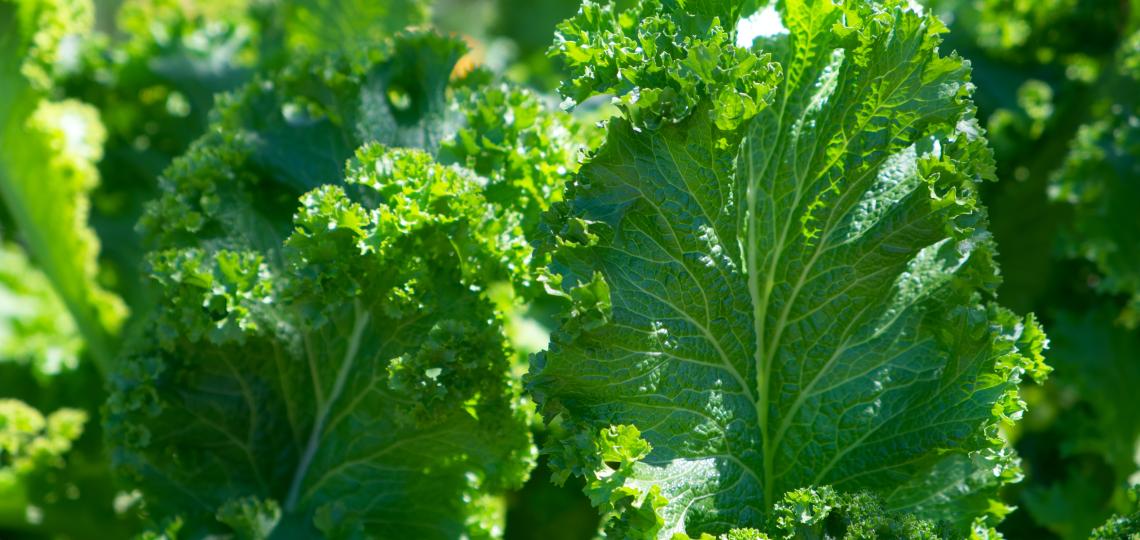
Defining a pathway of oxalic acid catabolism and its influence on the nutritional composition and phytochemical profile in dietary important plants
Dietary oxalic acid, consumed primarily through plant-based foods, has been shown to contribute to the formation of kidney stones, decrease the nutritional value of edible plants by acting as an antinutrient, and even exacerbate symptoms associated with other conditions. The incidence of these negative impacts appears to be rising and will most likely continue to rise with the recommendations for an increase in the consumption of fruits and vegetables. Although dietary oxalic acid has been known to have a negative impact on human health our understanding of the mechanisms regulating its concentration in plant foods is lacking.
Recently, we identified an oxalyl-CoA synthetase that is responsible for catalyzing the first step in a previously uncharacterized pathway of oxalate degradation in the plant model Arabidopsis. In this project we will extend this initial finding by first assessing whether this novel pathway of oxalate degradation plays a role in regulating the concentration of oxalate found in selected leafy greens.
Second, we will identify and characterize the genes and encoded enzymes responsible for catalyzing each step in this pathway of oxalate catabolism.
Third, we will determine the influence of this pathway of oxalate catabolism on the nutritional composition, phytochemical profile, and production characteristics in selected leafy greens.
It is anticipated that completion of the proposed basic and applied objectives will result in a gain in knowledge and resources beneficial to a broad range of scientists, producers interested in growing a more healthful food supply, and diet conscious consumers.
Supported by USDA, ARS, CRIS 3092-51000-061-001S
Factors regulating plant nutritional quality and productivity
The increasing frequency of abiotic stress, commonly associated with climate change, negatively impacts the nutritional quality and productivity of crop plants (e.g., legumes), and thus, has become a major threat to global food security. In response to this threat plant scientists have been trying to establish legumes that maintain good productivity under conditions of abiotic stress. To date, plants engineered with abiotic tolerance, in general, also exhibit penalties in yield and often are compromised in other aspect of growth and development. Thus, the development of new strategies that minimize these penalties are required. In addition to yields, the impact of these genetic modifications on nutrient concentrations, before and after exposure to abiotic stress, should also be considered. Likewise, effort to engineer nutritionally improved plants need to consider issues of reduced plant productivity and other pleotropic effects under abiotic stress environments. In this study, gene targets with the potential to confer abiotic tolerance (with lower penalties) as well as gene targets with the potential to confer an enhancement in nutritional quality (with lower penalties) will be selected and genetically modified in the model legume, Medicago truncatula. Plants containing the selected gene modification then will be assessed for changes in nutritional quality and productivity under abiotic stress and non-stress conditions. Plant lines displaying abiotic tolerant traits then will be crossed to the engineered plants containing nutritional improvements to generated plant lines containing both desired traits. Plant lines containing these stacked traits will also be evaluated for changes in nutritional quality and productivity under stress and non-stress conditions. Investigation into the mechanisms conferring the abiotic and nutritional improvement will also be initiated using molecular-genetic methodologies. This information is necessary for the design of rational translational strategies to help meet our future global food and nutritional needs.
Supported by USDA
Structural and mechanistic studies of oxalate catabolism
Oxalic acid is biosynthesized by different organisms to gain a selective advantage to enhance their survival. Considering the widespread occurrence of oxalate in nature and its broad impact on a host of organisms, it is surprising that so little is known about the turnover of this important acid. Recently, we discovered a CoA-dependent pathway of oxalate catabolism and found that it is present in both plants and microbes, underscoring the broad importance of this pathway. As a step towards elucidating this CoA-dependent pathway of oxalate catabolism, we propose to conduct structural and functional studies to gain a better understanding of the biological role and mechanism of key enzymes in oxalate turnover. Such studies will provide a starting point for the rational manipulation of the substrate and product specificities of these key enzymes and facilitate metabolic engineering of plants directed toward improving the nutritional quality and production of plant derived foods.
Supported by NSF
Deciphering the mechanism of calcium oxalate crystal formation and function
The biologically controlled formation of mineral deposits is often referred to as biomineralization. Biomineralization is widespread in nature occurring in a range of organisms from the simple microbe to complex humans. Understanding the process of how living organisms biosynthesize these intricate and biomolecular materials could have a beneficial impact on nutrition, agriculture, medicine, engineering, climate science, and material science. Although calcium oxalate crystal formation is the most common biomineralization process in plants, very little is known about how and why these crystals form. The diversity of crystal morphologies, as well as their prevalence and spatial distribution have led to a number of hypotheses regarding the biological function of crystal formation in plants. Proposed functions include roles in ion balance, in plant defense, in tissue support, in detoxification, and in light gathering and reflection. Definitive proof validating many of these hypotheses are still lacking. As a step toward determining how and why plants form crystals of calcium oxalate, we established an integrated molecular-genetic approach to deconstruct this process in the legume model, Medicago truncatula and reconstruct this process in Arabidopsis.